Revolutionary Breakthroughs: Top 05 Modern Medical Research Innovations Transforming Healthcare
What is the Modern Medical Research
In the rapidly evolving world of healthcare, modern medical research has led to groundbreaking discoveries that not only save lives but also improve the quality of life for millions. With advancements in technology, genetics, and pharmaceutical research, we’re witnessing revolutionary changes in how diseases are diagnosed, treated, and even prevented. Let’s dive into the top 10 modern medical research breakthroughs that are shaping the future of medicine.
Table of Contents
1. CRISPR and Gene Editing
In recent years, CRISPR (Clustered Regularly Interspaced Short Palindromic Repeats) has revolutionized the world of genetics and biomedical medical research. It’s a groundbreaking technology that allows scientists to edit genes with unprecedented precision. The applications range from curing genetic diseases to improving crop yields, but with such power also comes great ethical responsibility.
Let’s dive into what CRISPR and gene editing really mean, how they work, and the profound implications they hold for the future.
What is CRISPR?
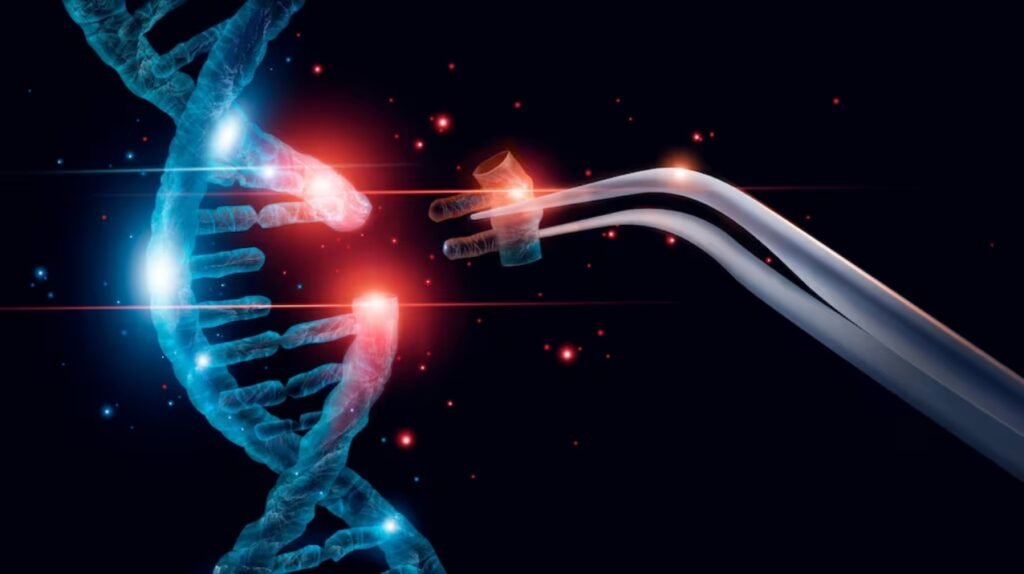
CRISPR is a naturally occurring system found in bacteria. It serves as a defense mechanism against viruses. When a virus attacks a bacterium, the bacterium stores a small piece of the virus’s DNA in its own genome. If the same virus tries to attack again, the bacterium uses this stored DNA as a guide to recognize and destroy the invader. This system gave scientists the idea to use CRISPR as a tool to target specific sequences of DNA in any organism, including humans.
The key component of this technology is Cas9, an enzyme that acts like molecular scissors. Once CRISPR identifies a target DNA sequence, Cas9 can cut the DNA at that precise location, allowing scientists to either disable a gene or insert a new one.
How Does CRISPR Work?
The process is remarkably simple for such a powerful tool in Medical Research:
- Guide RNA: Researchers design a piece of RNA (the guide RNA) that matches the DNA sequence they want to edit. This acts like a GPS for the Cas9 enzyme.
- Cas9 Enzyme: Cas9 is then introduced into the cells, and the guide RNA directs it to the exact location of the target gene.
- DNA Cutting: Once the Cas9 finds the right spot, it makes a cut in the DNA.
- Gene Editing: After the DNA is cut, the cell’s natural repair machinery either tries to repair the cut, which can disable the gene, or scientists can introduce new genetic material to be incorporated into the DNA sequence.
This mechanism is not only fast and cost-effective but also highly accurate, making it a preferred method for gene editing.
2. Immunotherapy for Cancer
Cancer, a disease that has touched almost every family in some way, continues to be one of the leading causes of death worldwide. Over the years, we’ve seen groundbreaking innovations in cancer treatment, from surgery and radiation to chemotherapy. Yet, one area that’s truly revolutionizing how we fight cancer today is immunotherapy—a treatment that harnesses the power of the body’s own immune system to combat cancer cells. This article explores how immunotherapy works, its types, benefits, and the promising future it holds in cancer care.
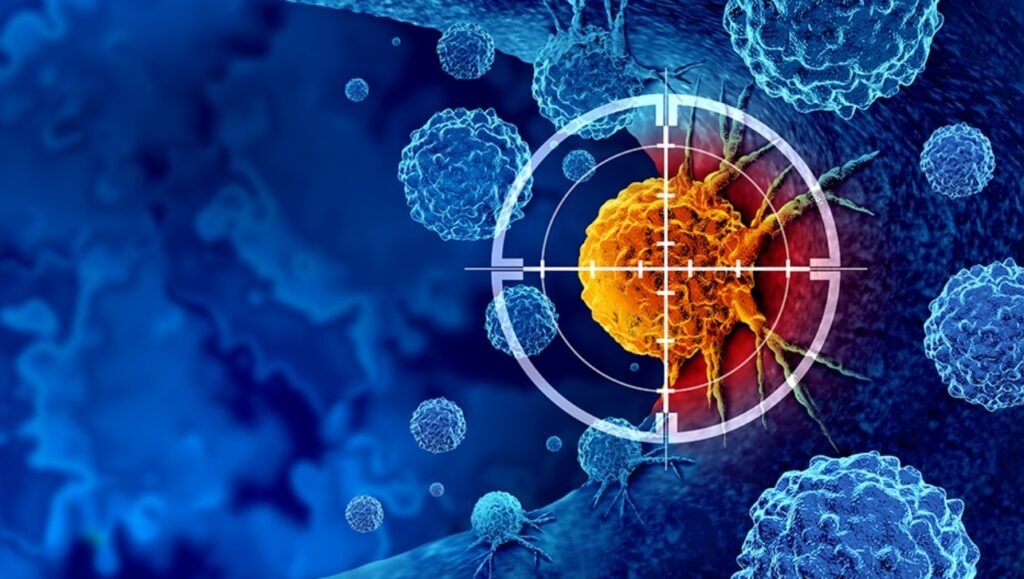
What is Immunotherapy?
Immunotherapy, at its core, is a type of cancer treatment that helps your immune system fight cancer. The immune system, a network of cells and proteins, protects the body against infections and diseases, including cancer. However, cancer cells can sometimes avoid detection by the immune system, allowing them to grow unchecked. Immunotherapy works by either boosting the immune system’s ability to recognize and attack cancer cells or by introducing components like antibodies to target cancer directly.
Types of Immunotherapy
Immunotherapy isn’t a one-size-fits-all treatment. It comes in several forms, each working in different ways to enhance the body’s immune response:
1. Checkpoint Inhibitors: These drugs prevent cancer cells from turning off the immune response. Certain cancer cells use proteins to blind immune cells, allowing the cancer to grow unchecked. Checkpoint inhibitors block this interaction, helping immune cells attack cancer more effectively.
Example drugs: Pembrolizumab (Keytruda), Nivolumab (Opdivo)
2. CAR T-cell Therapy: In this personalized treatment, a patient’s T cells (a type of immune cell) are taken from their body, genetically engineered in a lab to better attack cancer cells, and then reintroduced into the patient’s bloodstream. It’s like giving your immune system a powerful upgrade.
Example: CAR T-cell therapy for certain types of lymphoma and leukemia
3. Cancer Vaccines: Unlike traditional vaccines, which prevent diseases, cancer vaccines aim to treat existing cancer. These vaccines stimulate the immune system to recognize specific cancer proteins and fight the disease.
Example: Sipuleucel-T (Provenge) for prostate cancer
4. Monoclonal Antibodies: These lab-made proteins can either boost the immune system’s ability to fight cancer or directly attack cancer cells by marking them as targets for the immune system.
Example: Rituximab (Rituxan), often used in blood cancers
Benefits of Immunotherapy
Immunotherapy offers several advantages over conventional treatments, making it an attractive option for many cancer patients.
1. Targeted Action: Unlike chemotherapy, which can harm healthy cells and cause side effects like hair loss and nausea, immunotherapy works more precisely, targeting only cancer cells.
2. Durability of Response: Some patients treated with immunotherapy experience long-lasting remission. Once the immune system is trained to fight the cancer, it may continue to do so for years, even after treatment stops.
3. Broad Application: Immunotherapy has shown promise in treating a variety of cancers, including melanoma, lung cancer, bladder cancer, and certain blood cancers. Research continues to expand its applicability.
4. Less Toxicity: While immunotherapy can cause side effects, they are often less severe compared to those seen with chemotherapy or radiation, making it a more tolerable option for many patients.
Challenges and Side Effects
While immunotherapy has shown incredible promise, it’s not without its challenges medical research:
1. Not for Everyone: Immunotherapy doesn’t work for all cancer patients. Some tumors respond exceptionally well, while others don’t seem to be affected by immunotherapy. Researchers are working to understand why this is the case in modern medical research.
2. Immune-Related Side Effects: Since immunotherapy boosts the immune system, it can sometimes cause the immune system to attack healthy tissues, leading to autoimmune-like side effects. Common side effects include inflammation of the lungs, liver, or other organs. However, these side effects are generally manageable.
3. Cost: Immunotherapy treatments can be expensive, especially since they are often personalized. However, ongoing research and the development of new therapies may help reduce costs in the future medical research .
3. mRNA Vaccines
Vaccines have long been a cornerstone of public health, protecting humanity from deadly diseases like smallpox, polio, and measles. However, the introduction of mRNA vaccines has marked a revolutionary moment in the field of immunization. This new technology in medical research, which rose to prominence during the COVID-19 pandemic, offers hope not just for battling the coronavirus but also for many other diseases in the future. So, what exactly are mRNA vaccines, how do they work, and why are they considered a game changer in medical research?
What is mRNA?
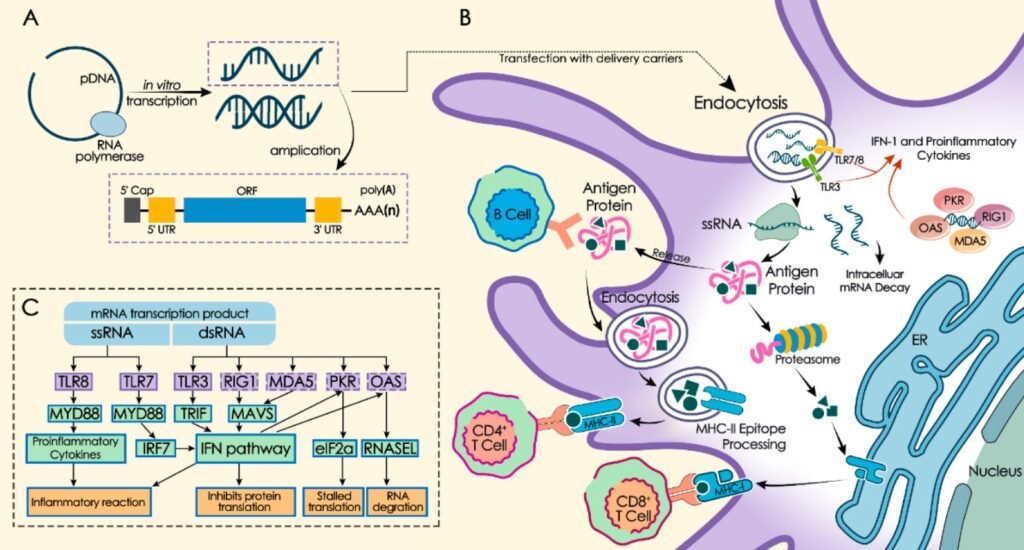
To grasp the concept of mRNA vaccines, it’s essential to understand messenger RNA (mRNA). RNA, or ribonucleic acid, is a type of molecule that plays a crucial role in the coding, decoding, and expression of genes. Specifically, mRNA carries instructions from DNA to the cell’s machinery for producing proteins. In simpler terms, think of mRNA as a messenger delivering a recipe from the genetic blueprint (DNA) to the kitchen (cell), where proteins, the building blocks of life, are made.
How Do mRNA Vaccines Work?
Traditional vaccines usually introduce a weakened or inactive virus into the body, prompting the immune system to recognize and combat the real virus if it ever appears. mRNA vaccines, on the other hand, take a different approach. Instead of introducing a virus, they introduce a small piece of genetic code, the mRNA, that instructs cells to produce a protein that is similar to part of the virus—usually the spike protein of the virus (like in the case of COVID-19).
Here’s a breakdown of how mRNA vaccines work:
- Injection of mRNA: When you receive an mRNA vaccine, it contains mRNA that encodes for a specific viral protein (e.g., the spike protein of the coronavirus).
- Protein Production: Once inside your body, the mRNA is absorbed by cells, where it provides instructions to produce the viral protein.
- Immune Response Activation: The immune system recognizes this protein as foreign and mounts an immune response by producing antibodies. If the real virus ever infects the body in the future, the immune system remembers how to fight it.
Key Benefits of mRNA Vaccines
- Speed of Development: mRNA vaccines can be designed and produced much faster than traditional vaccines. For instance, once the genetic sequence of the coronavirus was known, companies were able to develop and test mRNA vaccines in a matter of months.
- Flexibility: mRNA vaccines are highly adaptable. Scientists can adjust the genetic sequence within the mRNA relatively easily, which is useful for targeting new variants of a virus or different diseases altogether.
- No Risk of Infection: Since mRNA vaccines do not use live or inactive viruses, there is no risk of causing disease from the vaccine itself.
- Potential Beyond COVID-19: mRNA technology has opened doors to develop vaccines for a range of diseases, including influenza, Zika virus, and even some forms of cancer. Researchers are exploring ways to harness mRNA technology for targeted cancer treatments by instructing the immune system to attack cancer cells specifically.
Myths and Concerns About mRNA Vaccines
Despite their promise, mRNA vaccines have also been the subject of misinformation and concerns. Here are a few common misconceptions:
- “mRNA vaccines alter your DNA”: This is not true. mRNA does not enter the nucleus of the cell where your DNA is housed. It only provides instructions to the cell’s machinery for making proteins and is quickly broken down by the body after the job is done.
- “mRNA vaccines were developed too quickly to be safe”: While the timeline for COVID-19 vaccines was unprecedented, the underlying technology for mRNA vaccines had been in development for decades. COVID-19 provided the impetus and funding to fast-track the process, but the vaccines still went through rigorous clinical trials and regulatory approvals.
- “Long-term effects of mRNA vaccines are unknown”: Like all medical products, long-term studies are necessary, but the evidence so far suggests that mRNA vaccines are safe. The mRNA itself doesn’t stay in the body long, as it degrades after delivering its instructions.
4. Artificial Intelligence in Diagnostics
Artificial Intelligence (AI) is changing the face of healthcare, and one of the most exciting areas of transformation is diagnostics. Traditionally, medical diagnostics required human expertise, experience, and intuition. However, AI is now helping doctors identify diseases faster, more accurately, and with less human error. This leap in technology is improving patient outcomes, reducing costs, and making healthcare more accessible. But how is AI doing this? Let’s dive deeper into the role of AI in diagnostics, the challenges it faces, and the future it promises.
What is AI in Diagnostics?
AI in diagnostics refers to the use of machine learning, deep learning, and other advanced AI technologies to analyze medical data and assist in diagnosing diseases for medical research. This can range from analyzing medical images like X-rays and MRIs to examining patient histories and lab results. AI algorithms can sift through massive datasets, identify patterns that are too complex for the human eye to see, and provide doctors with actionable insights.
Applications of AI in Diagnostics
1. Medical Imaging in medical research
One of the most well-known applications of AI in diagnostics is medical imaging. AI algorithms can process images from CT scans, MRIs, mammograms, and X-rays to detect abnormalities such as tumors, fractures, or lesions. For example, AI has shown remarkable accuracy in detecting early-stage cancers, such as breast cancer, where early diagnosis is critical. The technology can highlight areas of concern and assist radiologists in making more informed decisions.
2. Pathology
AI is also making its mark in pathology, where it assists in analyzing tissue samples. Traditionally, pathologists would examine these samples under a microscope. Now, AI tools can be trained to recognize cancerous cells, providing faster and sometimes more accurate diagnoses than human eyes alone. AI algorithms have even been used to predict how a patient might respond to certain treatments based on the molecular structure of the cells.
3. Genomics
In the field of genomics, AI is helping researchers identify genetic markers for diseases. By analyzing vast amounts of genetic data, AI can spot mutations or patterns that indicate a higher risk for diseases like Alzheimer’s, heart disease, or certain cancers. This application of AI opens the door to more personalized medicine, where treatments can be tailored to the individual’s genetic makeup.
4. Cardiology
AI is making strides in cardiology by analyzing electrocardiograms (ECGs), echocardiograms, and other heart-related data to predict and diagnose heart diseases. AI can quickly flag irregular heartbeats, valve issues, and other abnormalities, often catching problems that might go unnoticed by traditional diagnostic methods.
5. Ophthalmology
In eye care, AI is being used to screen for diseases like diabetic retinopathy, glaucoma, and age-related macular degeneration. AI algorithms can detect minute changes in the retina, allowing for earlier intervention and treatment, which is crucial for preventing vision loss.
Benefits of AI in Diagnostics
1. Improved Accuracy in medical research
One of the most significant advantages of AI in diagnostics is the potential for improved accuracy. By leveraging large datasets and learning from thousands of cases, AI systems can reduce human error, misdiagnoses, and missed diagnoses. This is particularly beneficial in fields like oncology, where early detection can be the difference between life and death.
2. Faster Results
AI can process data much faster than humans. For instance, while a radiologist may take time to analyze a series of scans, AI can perform the same task in a fraction of the time. Faster diagnoses mean faster treatment decisions, which can improve patient outcomes, especially in critical cases.
3. Accessibility
AI in diagnostics has the potential to make high-quality healthcare more accessible. In remote or underserved areas where specialists may not be available, AI can assist general practitioners in diagnosing complex conditions, ensuring patients receive timely care. This can help bridge the gap between rural and urban healthcare services.
4. Cost Reduction
AI can also reduce the cost of healthcare by streamlining processes, reducing the need for unnecessary tests, and shortening hospital stays. By catching diseases early and reducing human error, AI can help avoid costly complications and prolonged treatments.
5. 3D Printing of Organs and Tissues
In the world of healthcare, the dream of being able to create functional, living organs and tissues has long been seen as futuristic and nearly impossible. However, with the advancement of 3D printing technology, that dream is rapidly turning into a reality. The use of 3D printing in medicine, especially for organs and tissues, is revolutionizing the way we think about treatments and surgeries.
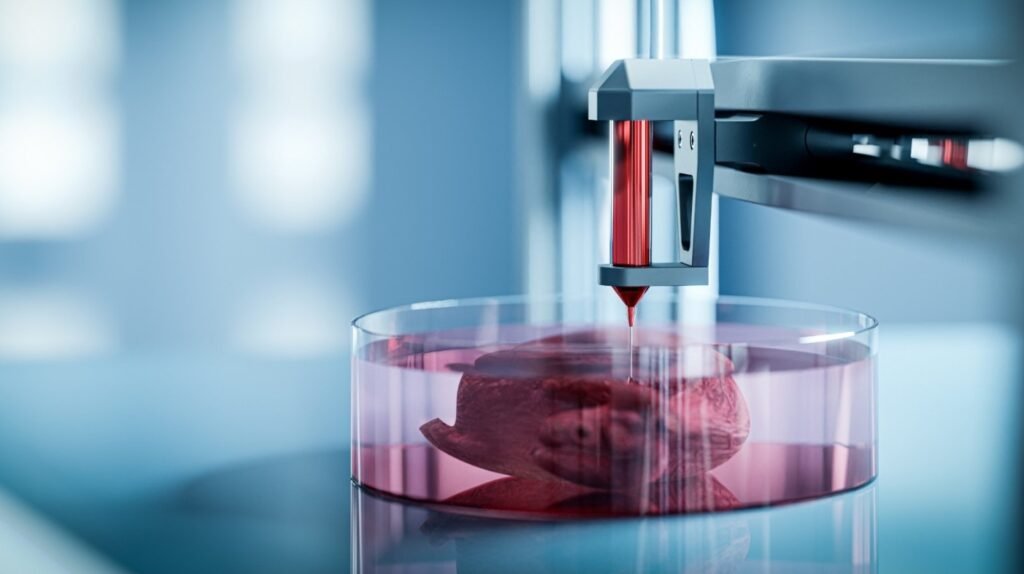
This technology not only promises to save lives but also to significantly improve the quality of life for millions of people around the world. Let’s dive deeper into how 3D printing is transforming the field of regenerative medicine.
What is 3D Bioprinting?
3D bioprinting is the process of creating three-dimensional structures, layer by layer, using living cells as the “ink.” Traditional 3D printing uses plastics or metals to build objects, but in bioprinting, bio-inks composed of living cells and biomaterials are used to fabricate tissues or even whole organs.
These bio-inks are loaded into a printer, and the cells are deposited in precise patterns to mimic the natural architecture of human tissue. Over time, these printed structures can develop into functioning tissues and, potentially, complete organs.
The Process of 3D Printing Organs and Tissues
The process of 3D printing organs and tissues can be broken down into several key steps:
- Imaging and Designing: Doctors start by using MRI or CT scans to create highly detailed digital models of the organ or tissue that needs to be printed. This ensures the printed structure is a perfect match for the patient’s anatomy.
- Bio-ink Preparation: Scientists harvest the patient’s own cells (or compatible donor cells) and culture them in the lab. These cells are then mixed with a biocompatible material, often hydrogels, to create bio-ink. The choice of bio-ink is critical because it needs to support the growth and development of the cells after printing.
- Layer-by-Layer Printing: The 3D printer, guided by the digital model, prints the tissue layer by layer, placing the bio-ink in precise locations. Depending on the complexity of the structure, this step can take hours or even days.
- Post-printing Maturation: After printing, the structure is placed in a bioreactor, where it is kept under optimal conditions (temperature, oxygen levels, nutrients) to allow the cells to grow and integrate with each other, forming functional tissue.
- Implantation or Testing: The printed tissue can either be used for research and testing, such as drug trials, or, in some cases, implanted into patients as a treatment.
3D Printing of Organs and Tissues: A New Frontier in Medical Research Healthcare
In the world of healthcare, the dream of being able to create functional, living organs and tissues has long been seen as futuristic and nearly impossible. However, with the advancement of 3D printing technology, that dream is rapidly turning into a reality. The use of 3D printing in medicine, especially for organs and tissues, is revolutionizing the way we think about treatments and surgeries.
This technology not only promises to save lives but also to significantly improve the quality of life for millions of people around the world. Let’s dive deeper into how 3D printing is transforming the field of regenerative medicine.
What is 3D Bioprinting?
3D bioprinting is the process of creating three-dimensional structures, layer by layer, using living cells as the “ink.” Traditional 3D printing uses plastics or metals to build objects, but in bioprinting, bio-inks composed of living cells and biomaterials are used to fabricate tissues or even whole organs.
These bio-inks are loaded into a printer, and the cells are deposited in precise patterns to mimic the natural architecture of human tissue. Over time, these printed structures can develop into functioning tissues and, potentially, complete organs.
The Process of 3D Printing Organs and Tissues in Medical Research
The process of 3D printing organs and tissues can be broken down into several key steps:
- Imaging and Designing: Doctors start by using MRI or CT scans to create highly detailed digital models of the organ or tissue that needs to be printed. This ensures the printed structure is a perfect match for the patient’s anatomy.
- Bio-ink Preparation: Scientists harvest the patient’s own cells (or compatible donor cells) and culture them in the lab. These cells are then mixed with a biocompatible material, often hydrogels, to create bio-ink. The choice of bio-ink is critical because it needs to support the growth and development of the cells after printing.
- Layer-by-Layer Printing: The 3D printer, guided by the digital model, prints the tissue layer by layer, placing the bio-ink in precise locations. Depending on the complexity of the structure, this step can take hours or even days.
- Post-printing Maturation: After printing, the structure is placed in a bioreactor, where it is kept under optimal conditions (temperature, oxygen levels, nutrients) to allow the cells to grow and integrate with each other, forming functional tissue.
- Implantation or Testing: The printed tissue can either be used for research and testing, such as drug trials, or, in some cases, implanted into patients as a treatment.
Applications of 3D Bioprinting in Medicine
The potential applications of 3D bioprinting in medicine are vast and groundbreaking:
1. Organ Transplants
One of the most exciting possibilities is printing entire organs for transplantation. Organ shortages are a global issue, with thousands of people dying each year while waiting for a donor. 3D bioprinting could provide a solution by printing organs tailored to the patient’s body, eliminating the need for donors and reducing the risk of organ rejection.
While we’re still a few years away from printing fully functional organs like hearts or kidneys, researchers have already successfully printed simpler tissues like blood vessels and skin.
2. Tissue Regeneration
Another promising application is in tissue regeneration. For example, burn victims or people with severe wounds could benefit from 3D-printed skin grafts made from their own cells. This would not only speed up healing but also reduce scarring and the risk of infection.
3. Drug Testing and Research
3D-printed tissues are also being used in pharmaceutical Medical Research to test new drugs. Traditionally, drug testing has been done on animals or on 2D cell cultures, which don’t accurately mimic human tissues. With 3D-printed tissues, researchers can create models that more closely resemble the human body, leading to more accurate results and reducing the need for animal testing.
4. Personalized Medicine
The ability to print tissues that match the unique biology of a patient opens the door to personalized medicine. Treatments could be tailored to the specific needs of each patient, resulting in more effective therapies with fewer side effects.
Challenges in 3D Printing of Organs and Tissues
While the promise of 3D bioprinting is huge in Medical Research, there are still significant challenges to overcome:
- Complexity of Organs: Simple tissues like skin or cartilage are relatively easy to print, but more complex organs like the heart or liver are far more difficult. These organs consist of multiple cell types, complex structures, and intricate blood vessel networks, all of which must be replicated for the organ to function properly.
- Vascularization: One of the biggest challenges in bioprinting is creating the blood vessel networks needed to supply oxygen and nutrients to large tissues or organs. Without a reliable vascular system, printed organs cannot survive after transplantation.
- Cell Source and Biocompatibility: Finding the right source of cells is another hurdle. Using a patient’s own cells is ideal to avoid immune rejection, but harvesting and culturing these cells can be time-consuming. Additionally, ensuring that the bio-inks are biocompatible and safe for the body is critical.
- Regulatory and Ethical Concerns: As with any new Medical Research technology, there are ethical and regulatory questions to address. How should printed organs be tested? Who owns the rights to these bioprinted tissues? These are questions that regulators and society as a whole will need to answer in the coming years.
The Future of 3D Bioprinting in Medical Research
The future Medical Research of 3D bioprinting is bright, with the potential to change the way we approach everything from surgery to drug development. While we may still be years away from seeing fully functional printed organs widely available, progress is being made every day. Researchers are working tirelessly to solve the current challenges and bring this technology to the forefront of medical treatments and Medical Research.
In the near future, we can expect to see bioprinting used more extensively in areas like tissue engineering, personalized medicine, and drug testing. The hope is that one day, organ shortages and long transplant waitlists will be a thing of the past, and patients will have access to custom-made organs created specifically for them.
05 Essential Health Gadgets for a Healthier Lifestyle
इन 10 चीजों से करें “पेट की चर्बी” को जड़ से खत्म-
World’s Top 5 Research Breakthroughs That Are Changing Our Future
6 comments